Researchers worldwide are speeding up particles in colliders and digging deep underground to find axions or WIMPs. No, these are not superhero codenames – but hypothetical particles that are some of the proposed candidates for dark matter. What is dark matter? Why is it worth studying? And how can scholars see the unseen? Let’s find out with Dmitry Glazov, a senior researcher at ITMO’s Faculty of Physics.
Credit: sakkmesterke / photogenica.ru
What is dark matter?
Dark matter is a hypothetical form of matter that appears to exist in space. It has never been registered nor directly observed by researchers.
It is called “dark” not because of its color, but because it is invisible to the eye. In fact, dark matter is transparent since it doesn’t radiate or interact with light. Let’s take a cup as an example. It consists of positively and negatively charged particles – also known as protons and electrons – which attract one another and stick together due to their electromagnetic properties. Therefore, the cup doesn’t fall apart and can reflect light, making it visible to us. Dark matter, on the other hand, is different. Since it has no bonding electromagnetic characteristics, scientists believe that it is evenly spread out in the universe; it doesn’t interact with light and thus can’t be spotted.
At the same time, dark matter builds up a range of cosmic phenomena such as stars and galaxies with the help of gravity. For instance, it increases the rotation speed of stars at the periphery of galaxies, thus making them move as fast as those in the center of the galaxy.
How was it discovered?
Back in the 1930s, Fritz Zwicky, a Swiss astronomer, observed the rotations of spiral-shaped galaxies. According to the laws of mechanics, stars located at a galaxy’s edges must move slower than those in its middle, otherwise, they would fly apart. In fact, Fritz Zwicky found that all stars in a spiral galaxy travel at nearly equal speeds, whereas periphery stars move as if the galaxy was being held together by some invisible substance with a much greater mass. This mysterious substance, which can only be detected through its gravitational interaction with other objects, was called dark matter. In the 1970s, American astronomers Vera Rubin and Kent Ford revealed its presence in other spiral galaxies, particularly in the Milky Way. But even though there are more and more observations that dark matter exists, its nature still remains a mystery.
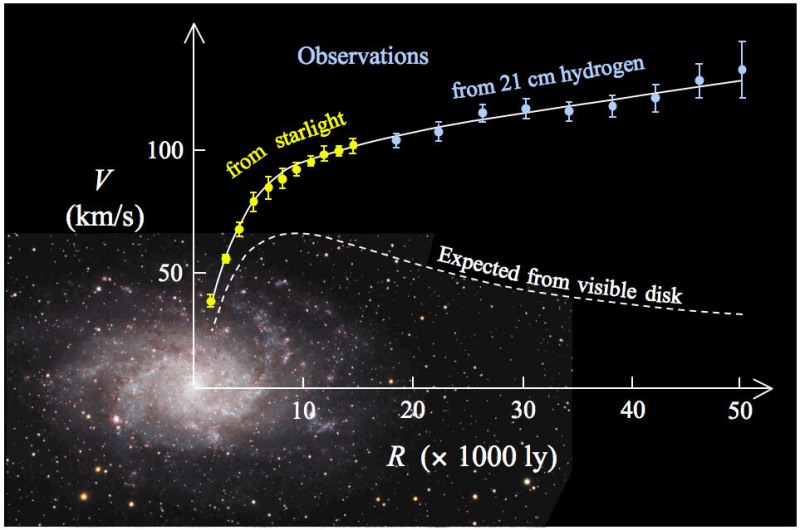
The actual rotation speed of galaxies (top) differs significantly from predictions made based on the presence of visible matter (bottom). Credit: Mario De Leo / Wikimedia Commons / CC-BY-SA-4.0
Why is it important?
Understanding dark matter can aid in explaining how the universe works. Particularly, physicists hope that it will help build a theory of everything – a hypothetical framework that will fully explain all physical phenomena, from elementary particles to galaxies and dark matter, and even predict new ones.
More specific implications of dark matter research are yet to be determined. Yet history has seen similar discoveries that have changed not only academia but also our daily lives. When French physicist Henri Becquerel discovered radioactivity in 1896, no one had any idea how to use it for the good. Nonetheless, investigations continued, and in 1934 Frédéric and Irène Joliot-Curie became the first to induce artificial radioactivity, transforming an isotope aluminum-27 into a radioactive isotope phosphorus-30.
Nowadays, artificial radioactivity is ubiquitous in a range of fields, including nuclear energy, X-ray diagnostics, and radiation therapy. It is also used for radiometric dating of objects and events in geology, paleontology, and archeology, and even for authenticating artworks. This could be the case with dark matter, too.
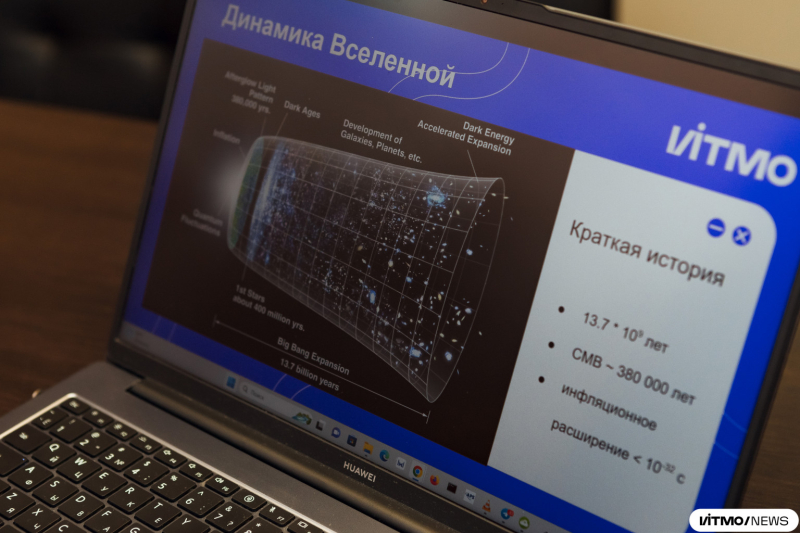
Photo by Dmitry Grigoryev / ITMO.NEWS
Current research
Mass maps of dark matter. For this, astrophysicists rely not only on galaxy rotation but also on gravitational lensing. Imagine you’re looking at a distant star through a telescope and see a glowing halo or split around it. This happens because the star’s light doesn’t directly enter the telescope but gets curved, interacting with different invisible objects along the way. As a result, the light travels around the object in an arc on both sides, which resembles the way lenses in, say, glasses, work, except that here this role is played by the object’s mass – dark matter for the most part. By knowing the number and mass of visible objects, the distance to the lens object and the observed star, and by calculating the degree of image deviation, scientists can calculate the distribution of dark matter mass.
Research shows that dark matter makes up over 80% of the universe, whereas visible matter – only 20%. These figures are not final – as observations are still ongoing. Recently, using the data from the Gaia telescope, researchers estimated the total mass of our galaxy to be half as much as previously thought. Notably, scientists track not only each space object individually, but also calculate overall statistics that can be extrapolated to the entire universe.
Composition of dark matter. There are several models that can explain the nature of dark matter, the two most prominent of them are:
-
Weakly interacting massive particles (WIMPs) are heavy particles that interact with one another exclusively through gravity. The term was first coined by American cosmologist Michael Turner in 1986.
-
Axions are light particles that were predicted by Nobel laureates Frank Wilczek and Steven Weinberg in the 1970s. Axions exhibit a weak interaction with ordinary matter and can convert to photons in a strong magnetic field.
Yet none of these hypothetical particles have been discovered or observed thus far.
Nevertheless, researchers around the world pursue various approaches to trap dark matter particles:
-
Some are trying to catch them using sensitive detectors located in underground laboratories. The idea is that all spaceborne particles, apart from dark matter particles, should be stopped by the Earth's thickness. Once in the laboratory, the particles are placed in a special cylinder containing a noble gas (xenon or argon). Inside, the particles interact with the gas, ionize it, and then disintegrate into visible particles – photons. All activities inside the cylinder are registered by the detector, which can identify if a particle is of dark matter nature based on the reaction characteristics.
-
Some are employing colliders – massive machines used to accelerate beams of charged particles, be it electrons, protons, or ions, nearly to the speed of light and drive them to smash with one another. Researchers believe that such collisions may result in as-yet undiscovered particles, in particular related to dark matter. In 2012, scientists confirmed the existence of the Higgs boson – an elementary particle that gives mass to other fundamental particles – through the experiments at the Large Hadron Collider (LHC) at CERN in Switzerland. The Higgs boson has become the final missing piece in the Standard Model that describes the microworld.
-
Others are performing precise experiments with particles in electromagnetic traps, where single electrons, atoms, and molecules can be held and controlled for extended periods of time. In doing so, researchers can detect an atom's characteristics, such as its magnetic moment, by measuring its vibrational frequencies with precision faster than one trillionth of one second. And with the help of contemporary quantum theory, they can calculate the magnetic moment just as accurately. If dark matter interacts with ordinary matter, even if only weakly, the magnetic moment should diverge from the anticipated value. By comparing theory and experiment, scientists can identify this difference and, consequently, discover how dark matter manifests itself.
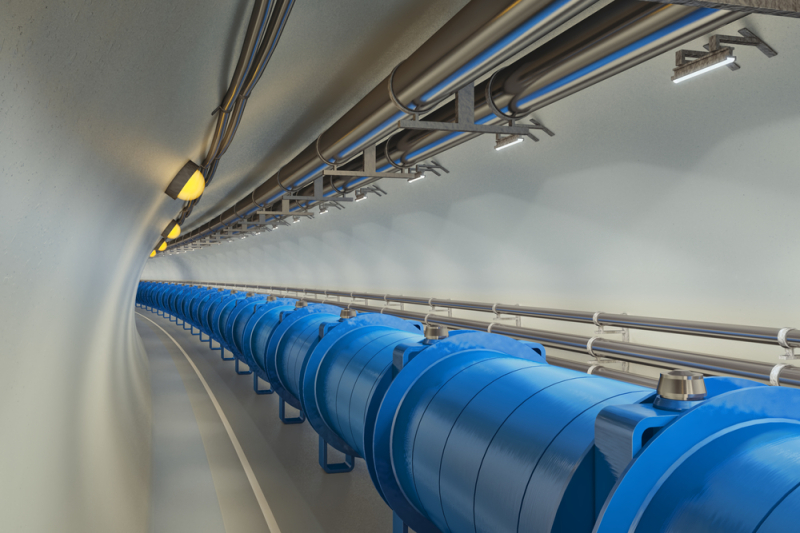
A hadron collider. Credit: sssccc / photogenica.ru
Dark matter studies at ITMO
There are several research groups at ITMO that deal with dark matter, one of which is a team led by Maxim Gorlach, an associate professor at the Faculty of Physics and the head of the Laboratory of Probing Fundamental Physics with Topological Metamaterials. The researchers are part of the international consortium searching for cosmic axions. Together with Frank Wilczek, the team has devised a metamaterial that may be used to investigate the properties of axions. Metamaterials areartificially engineered substances, the electromagnetic properties of which are defined by their chemical composition and structure. In the new metamaterial, quasiparticles exhibit the same properties as the hypothetical axions.
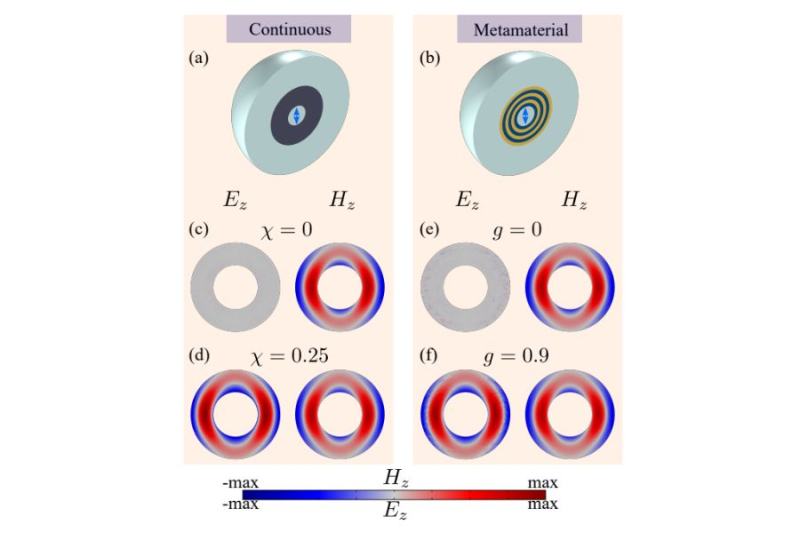
In this research, magnetic dipoles are placed in a shell of axions (left) and a metamaterial one (right). The study shows that the resulting electromagnetic field is the same in both cases, meaning that the metamaterial displays axion properties. Illustration from the article / Physical Review B
The research team of Andrey Volotka, a professor at ITMO’s Faculty of Physics, uses high-precision calculations of quantum electrodynamics diagrams to forecast the magnetic moment of atomic systems. This is a quantity that characterizes the magnetic properties of a substance or, in other words, its ability to generate and perceive a magnetic field. This property is critical for both electrons and other atoms. Theoretical physicists are continually calculating a more exact number with adjustments of a specific order, while experimental physicists validate their calculations with high-precision tests. Free electrons, for instance, have obtained fifth-order corrections as of now.
Calculations for atoms are far more complicated – yet yield more data, unlike experiments. The more exact the figures are, the more likely it is to spot a deviation, which may point to the existence of a new particle, particularly one connected to dark matter.
Alena Mamaeva
Journalist
Marina Belyaeva
Translator